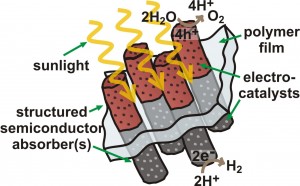
Materials chemist Shannon Boettcher and his lab are investigating ways to use non-carbon-based (i.e. “inorganic”) materials – like the semiconductors found in a computer or cell phone – to convert and store energy sustainably and in a cost-effective fashion. This research contributes to what Boettcher predicts will be a dramatic shift in energy technologies necessary to prevent substantial climate change. Currently, he and his colleagues are studying fundamental and practical aspects of the production of chemical fuels using water, sunlight and/or electricity. The stable chemical fuels they create, such as hydrogen gas (H2), can be easily stored and later used in fuel cells or burned like natural gas – a potentially much less expensive way to store energy than batteries.
In 2014 Boettcher and his (now former) graduate student Lena Trotochaud discovered that iron impurities in common materials were critical in the process that splits water molecules to make H2 and O2. Boettcher says, “We figured out that this iron contamination was happening, and while there was evidence of this in the literature, it wasn’t well understood. Lena developed a simple way to get every bit of iron impurity out and showed that catalysts that scientists had thought were good became a thousand-fold worse when the trace iron impurities were removed.” Boettcher’s findings stirred so much interest that two of his papers were at one time ranked in the top 0.1% of most frequently cited papers in his field. “This is actually some of the simpler work that we’ve done,” he says, “but it’s having a huge impact because it’s changed the paradigm of how people look at these materials.”
Boettcher finds that the most exciting part of his work is collaborating with students and fellow researchers. He says it is “important for the public to understand that although universities do important basic research that has applications and eventually a big impact on society, the tremendous short term return is the students that go through the program, and go off and work in, for example, high-tech companies. They work on the teams that invent the next iPhone or an improved solar cell – we help develop their scientific and problem-solving skills they need to succeed throughout their career.” Given the potential for growth in the field of materials, and the importance of alternative energy research for sustainability and the reduction of carbon emissions, Boettcher says that materials science research is a “great thing to be involved in, and I have a lot of excellent students, because they realize that.”
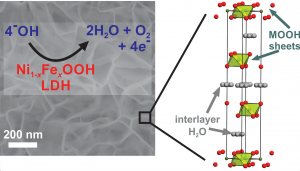